Physics: Particles not supposed to exist simulated in lab
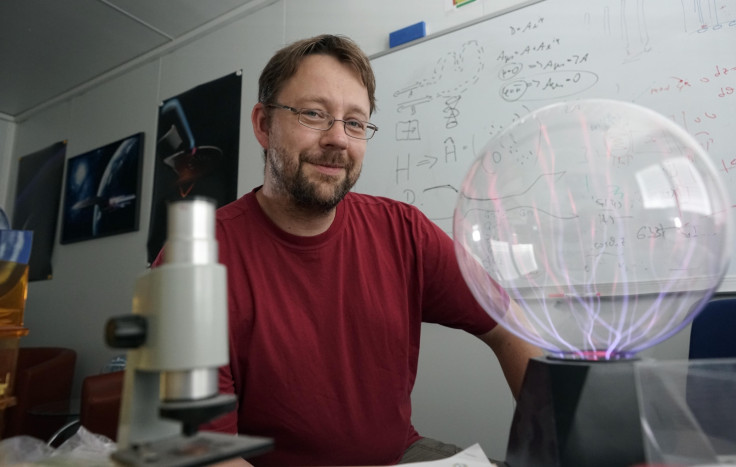
An international team of scientists has been able to simulate the mysterious, invisible particle Majorana, which cannot be observed normally. In fact, by virtue of its exotic nature, it cannot even exist in the physical world.
Together with colleagues from Technical University of Greece and Centre for Quantum Techologies Singapore, Alexander Szameit and his team at the Friedrich Schiller University Jena, Germany, developed a photonic set-up with complex waveguide circuits engraved in a glass chip, which enabled them to simulate charged Majorana particles.
Majoranas are a special kind of quasi-particle where the particle is its own antiparticle, but instead of annihilating each other, the Majorana particle stays neutral and interacts weakly with the environment, much like the neutrinos.
"The key idea is that the (charged) Majoranon can be decomposed into two Majorana fermions (uncharged solutions of the Majorana equation). The uncharged Majoranas follow the same Dirac equations as simulated by light in coupled waveguide lattices," the first author of the study Dr Robert Keil, formerly of Szameit's team and now with University of Innsbruck, Austria, told IBTimes UK.
The two rays of light sent through parallel running waveguide lattices show the opposing characteristics separately, he explained.
The two waves interfere to form a light distribution that corresponds to that of a Majorana particle wave function giving its position at a defined time.
"With the help of many of such single images the particles can be observed like in a film and their behaviour can be analysed," says Keil.
Light simulates equation
The evolution patterns of the Majoranon particles arise from the dynamics of the Majorana equation, which is merely being simulated by the interference of light, says Keil, stressing that the light only simulates the Majoranon, and not creating any particle.
This model allows access to phenomena that so far only have been described in exotic theories, by Professor Alexander Szameit of the Friedrich Schiller University Jena. "Majoranons are of an entirely theoretical nature and cannot be measured in experiments."
The system allows scientists to conduct non-physical processes that normally cannot be observed in nature, he adds.
The existence of Majoranons would violate conservation of charge. Therefore, they do most likely not exist -- Robert Keil
A non-physical process violates fundamental laws of physics. Energy-non-conservation would be such a process. Here, the existence of Majoranons would violate conservation of charge. Therefore, they do most likely not exist, as Keil puts it.
Szameit foresees application of simulated Majoranons in a new generation of quantum computers. "With this approach, much higher computing capacities than are possible at the moment can be achieved."
Quantum computing
In quantum computing, electrons are coaxed to represent states that are not only representative of ones and zeros of conventional computers but also a quantum superimposed state that is both a one and a zero. But interactions with the surrounding can cause such electrons to collapse into traditional states.
That is where the weakly interacting Majorana which is both matter and antimatter comes with an advantage.
Very few attempts to observe the Majorana have been successful with Delft University of Technology in the Netherlands in 2012 and Princeton scientists last year claiming to have sighted the fermion.
To that extent, the simulation achieved at Jena could be a better option.
Majoranas were first postulated in the 1930s by the young Italian scientist Ettore Majorana, who disappeared just like the mysterious particle he predicted was stable despite being both matter and antimatter.
Some scientists believe the elusive neutrinos are Majoranas while some others suspect Majoranas are what make dark matter, undetected so far.
© Copyright IBTimes 2024. All rights reserved.