Massive explosion from unknown source billions of light years away baffles astronomers
Gamma ray burst detected from 12 billion light years away - but no one knows what caused it.
David Coward, University of Western Australia
At 10:49pm Western Australian time on February 2 this year, cosmic gamma rays hit the Nasa satellite, Swift, orbiting the Earth. Within seconds of the detection, an alert was automatically sent to the University of WA's Zadko Telescope. It swung into robotic action, taking images of the sky location in the constellation Ophiuchus.
What emerged from the blackness, where nothing was seen before, was a rapidly brightening "optical transient", which is something visible in the sky for a brief period of time.
The event, named GRB170202, was a very energetic gamma ray burst (GRB). After less than a minute, the gamma rays switched off, and the GRB appeared as a brightening and then fading optical beacon.
The Zadko Telescope recorded the entire evolution of the optical outburst. During its biggest outburst, GRB170202 was equivalent in brightness to millions of stars shining together from the same location.
About 9 hours 42 mins after the GRB, the Very Large Telescope in Chile acquired the spectrum of the light from the optical afterglow.
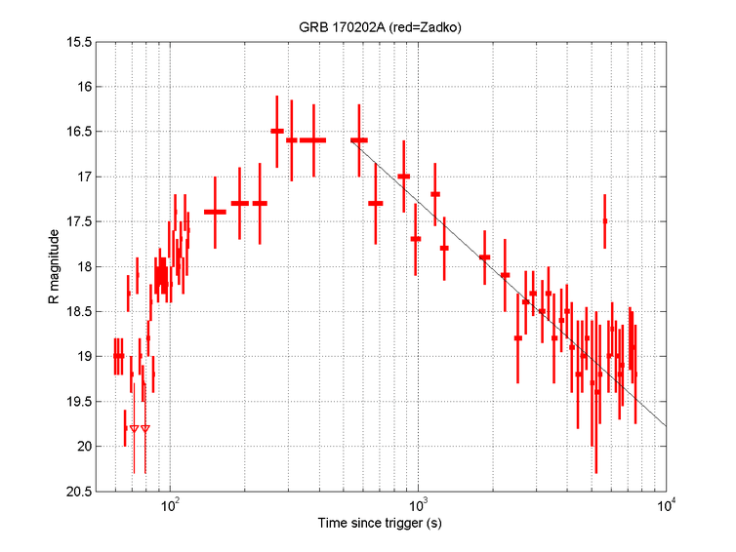
This enabled a distance to the burst to be measured: about 12 billion light years. The universe has expanded to four times the size it was then, 12 billion years ago, the time it took the light to reach Earth.
GRB170202 was so far away, even its host galaxy was not visible, just darkness. Because the GRB was a transient, never to be seen again, it is like turning on a light in a dark room (the host galaxy) and trying to record the detail in the room before the light goes out.
Mystery of gamma ray burst
The flash of gamma radiation and subsequent optical transient is the telltale signature of a black hole birth from the cataclysmic collapse of a star. Such events are rare and require some special circumstances, including a very massive star up to tens of solar masses (the mass of our Sun) rotating rapidly with a strong magnetic field.
These ingredients are crucial to launch two jets that punch through the collapsing star to produce the gamma ray burst (see animation). The closest analogue (and better understood transient) to a GRB is a supernova explosion from a collapsing star. In fact, some relatively nearby GRBs reveal evidence of an energetic supernova linked to the event.
Simulations show that most collapsing stars don't have enough energy to produce a GRB jet, a so-called "failure to launch" scenario. Both observation and theory show that GRBs are extremely rare when compared to the occurrence of supernovae.
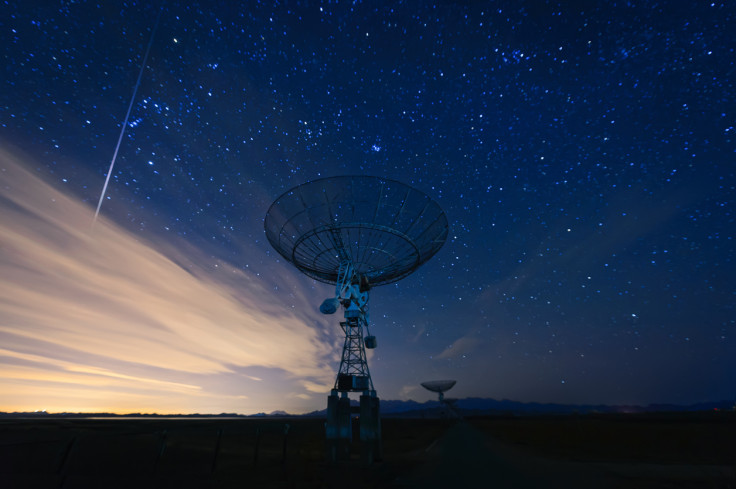
The stars that produce GRBs are born and die within some tens to hundreds of thousands of years, unlike our Sun which has been around for billions of years. This is because very massive stars exhaust their fuel very quickly, and undergo violent gravitational collapse leading to a black hole, on the timescale of seconds.
A plethora of rogue black holes
The rates of black hole formation throughout the universe can be inferred from the GRB rate. Based on the observed GRB rate, there must be thousands of black hole births occurring each day throughout the entire universe.
So what is the fate of these cosmic monsters? Most will be lurking in their host galaxies, occasionally devouring stars and planets.
Others will be in a gravitational death dance with other black holes until they merge into a single black hole with a burst of gravitational waves (GWs), such as the first discovery of such an event by the Laser Interferometer Gravitational-Wave Observatory (LIGO).
At the frontier of understanding black hole formation is the search for a special kind of GRB that marks the merger (collision) of two neutron stars. So called "short GRBs" are flashes of gamma radiation that last less than a second and could be the "smoking gun" for neutron star mergers.
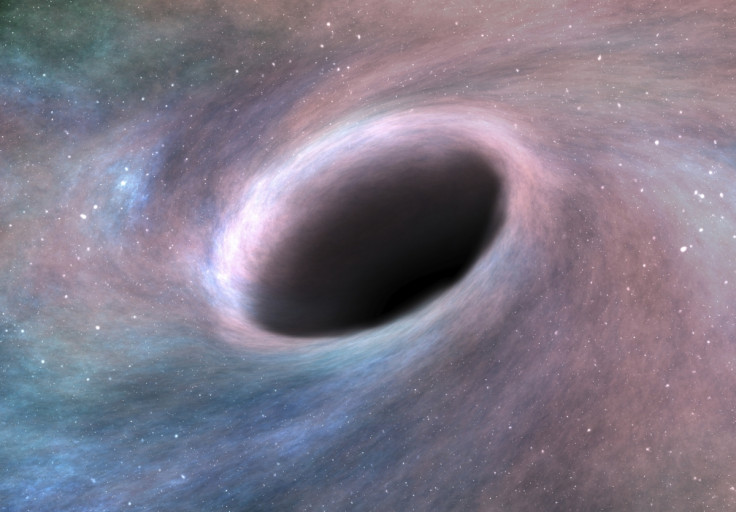
Importantly, merging neutron stars should be detected from their gravitational radiation by LIGO. Hence, a coincident detection in gamma rays, optical and gravitational waves is a real possibility.
This would be a monumental discovery allowing unprecedented insight into the physics of black hole formation. The revolution is like listening to the radio on a 1920s receiver and then watching a modern high definition surround sound movie.
Future challenges
Given the above rate of thousands of black holes created per day, it seems that coincident detection of GRBs and gravitational waves is a no brainer.
But in reality we must take into account the limited sensitivity of all the telescopes (and detectors). This reduces the potential observation rate to some tens per year. This is high enough to inspire a global scramble to search for the first coincident gravitational wave sources with electromagnetic counterparts.
The task is extremely difficult because the gravitational wave observatories cannot pinpoint the location of the source very well. To counter this, a strategy of searching for coincident gravitational wave and electromagnetic detections in time may be the best bet.
The newly funded ARC Centre of Excellence OzGrav mission is to understand the extreme physics of black holes.
One of the goals is to search for optical, radio and high energy counterparts coincident with gravitational waves from black hole creation. Australia is poised to play a significant role in this new era of "multi-messenger astronomy".
David Coward, Associate professor, University of Western Australia
This article was originally published on The Conversation. Read the original article.
© Copyright IBTimes 2025. All rights reserved.