Scientists succeed in levitating nanodiamonds in a vacuum using lasers
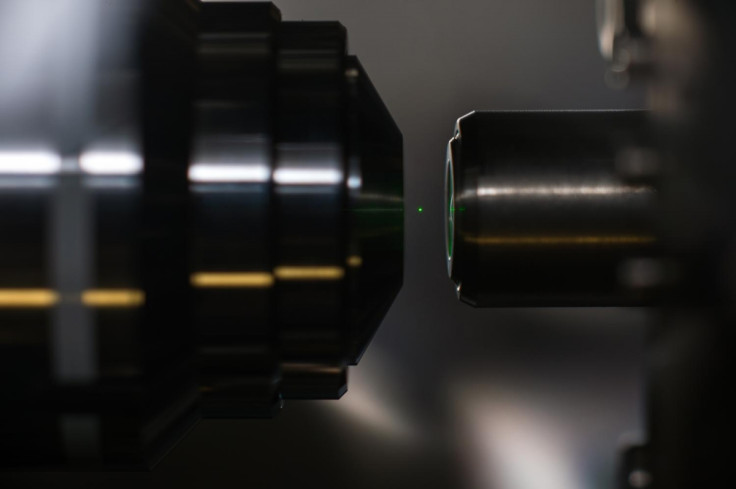
Scientists have succeeded in levitating nanodiamonds in a vacuum for the first time ever using a laser, making a physics breakthrough that could pave the way for a new breed of instruments able to sense tiny forces.
Researchers from the University of Rochester had previously discovered that it was possible to get nanodiamond particles to levitate in the air using a trapped laser, but their latest research proves that it is possible in vacuum too, which is the first step towards creating a "hybrid quantum system".
Nanodiamonds trapped at atmospheric pressure are continuously agitated by air molecules around them, but trapping them inside a vacuum removes this problem completely as the laser is able to pull on both microscopic and nanoscopic particles.
The research, entitled "Multi-dimensional single-spin nano-optomechanics with a levitated nanodiamond" is published in the journal Nature Photonics.
How does friction operate on tiny objects?
"This allows us to exert mechanical control over them. They turn into little harmonic oscillators," said Levi Neukirch, lead author of the paper and a PhD student at University of Rochester.
"We can measure the position of the diamond in 3D and we create a feedback signal based on the position and velocity of the nanodiamond. This lets us actively damp its motion."
In order make the nanodiamond particles stay levitating in the air, the researchers measured the tiny movements made by the particles and adjusted the intensity of the laser light. They built a system containing two lasers – one to excite the molecules so that the nitrogen vacancies emit light, which can then be measured, while a second laser then traps the molecules and levitates it in the vacuum.
"One of the reasons we're interested in this research is that the position of the crystal in the trap is a very sensitive probe of forces in its environment. Friction is a force we're very familiar with... but what we don't understand is what happens when we shrink objects down to very, very small scales, and how friction operates on objects," said Nick Vamivakas, an assistant professor with the University of Rochester's Institute of Optics who led the researchers.
"The reason this is important is because technology continues to shrink down to these length scales. We need to understand how the environment will interact with the devices that we're making."
Creating a macroscopic Schrödinger Cat state
The researchers next hope to reduce the oscillations of the nanodiamond particles down to absolutely zero – which is known as "ground state" – as this would make the system into a quantum mechanical oscillator.
At the moment, this is not yet possible, because low temperatures and pressures cause the nanodiamonds to either sublimate or melt, since at lower pressures there are fewer air molecules to remove excess heat from the diamonds and is caused by the laser during the experiment.
"Because the electron spins are intrinsically quantum mechanical, they can exist in something called superpositions. We can create a state where a single spin is in both the +1 and -1 states simultaneously," said Neukirch.
"If we can mechanically place the nanodiamond in the ground state, this would allow us to both push and pull on the spin, hopefully generating a mechanical superposition of the entire diamond. This is a curious phenomenon that physicists are interested in studying, and it is called a macroscopic Schrödinger Cat state."
© Copyright IBTimes 2025. All rights reserved.