Preparing for a quantum future: An inside look at groundbreaking research in Singapore
NUS is researching quantum encryption, quantum communications, atomic clocks and quantum thermodynamics.
What would a world with ultra-fast computers be like? The answer is, no one knows, because they don't exist.
There are lots of interesting use cases for quantum computers and other quantum technologies, and seeing as computer scientists have yet to even agree on which type of quantum computer will become the first proper prototype (light based? Trapped ions and atoms? Superconducting circuits?) everything else is just a theory for now.
But that doesn't stop researchers from considering lots of different ideas, and to that end, the National University of Singapore (NUS) is putting its eggs into lots of different baskets, so that whichever way quantum goes, hopefully they'll have it covered.
One key field of research is quantum encryption – the idea of what happens in future when a quantum computer is able to crack current RSA encryption methods, which rely on complex mathematical problems that are too difficult for a regular classical computer or a human to solve.
In theory, quantum computers will be able to factor impossibly large numbers very quickly, which would mean it could easily solve the mathematical puzzles used in the RSA public key and private key encryption method.
Quantum encryption
The director of NUS' Centre of Quantum Technologies (CQT) Artur Ekert founded the concept of quantum encryption a decade ago, and today CQT's researchers are trying to find a way to secure communications using light, so that no hacker can crack them.
The researchers worked out how to generate keys using light, known as quantum keys, but now they're trying to figure out how to transmit the light signals to different users, so that they can use the technology to secure their data – a field of research known as quantum key distribution (QKD).
"We generate a pair of photons that have a very strong correlation and we distribute them to different locations," CQT's principal investigator Dr Alexander Ling tells IBTimes UK.
"Traditionally, you need to have full trust in at least the part that is emitting the signal, that has to be under the control of either Alice or Bob. You must think of entanglement as a finite resource. The way we think of entanglement, if you only have two particles in the entanglement, they have a strong correlation, but if you had three parties, the entanglement correlation goes down.
"You reveal the presence of the eavesdropper in this way. This makes it possible for a third party to run the entanglement source and distribute photons to Alice and Bob, [who can then] measure the quality of the entanglement and determine that there's very likely to be no eavesdropping."
At the moment, it is difficult to transmit quantum keys over distances greater than about 40-50km.
"We're trying to expand the range of quantum key distribution. As you go over fibre, the photons have to transmit over glass, so we transmit the photons over air, and over a long distance we use satellites," said Ling.
The idea of using satellites to distribute quantum keys has been around for a long time, but satellites are hugely expensive to launch into space, and we will need a lot of them, if quantum encryption is to become a reality.
To get around this, NUS has focused on using tiny nanosatellites known as "cubesats" instead, and in December 2015, CQT became the first group in the world to demonstrate a quantum light source in space. But this is only the beginning, and research to improve the nanosatellite and the quality of its light source payload continues, with the aim of making the technology commercially viable.
CQT researchers, working together with Australia's RMIT University, have also proposed a way to operate a quantum computer in the cloud without exposing sensitive data or the software program being run, called "blind quantum computation".
Atomic clocks
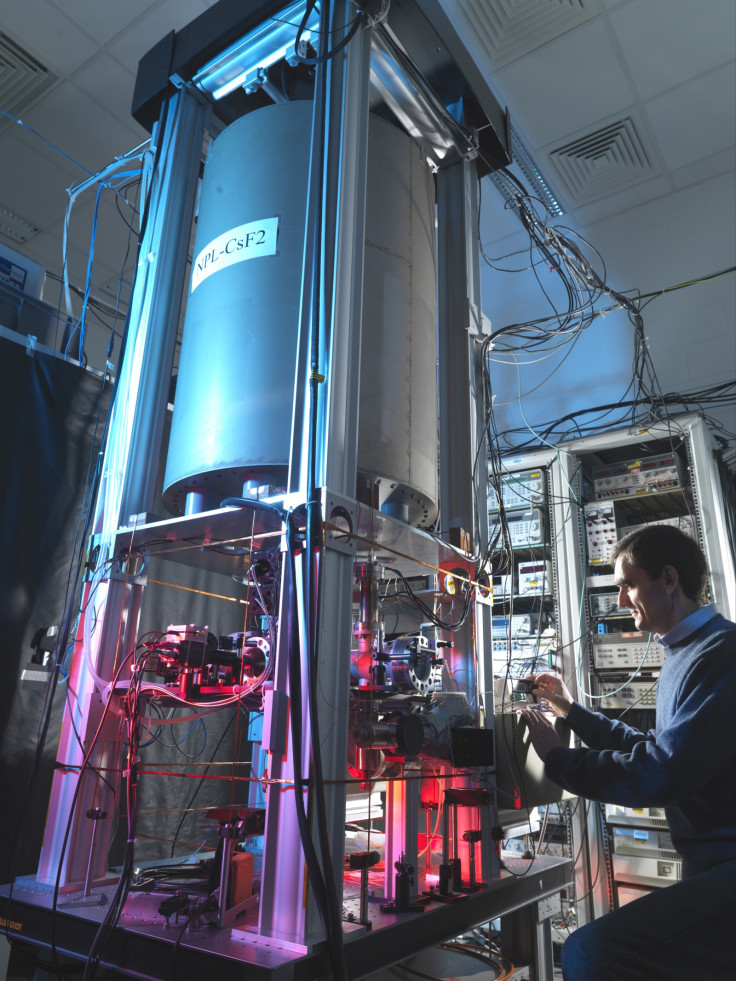
NUS is also trying to develop far more accurate clocks than we have today using quantum technologies, because the more accurate your clocks, the better you can measure things.
Currently, 500 microwave atomic clocks are used to calculate precise global time, from powering GPS satellite navigation, electric power grids and financial networks, to the mobile networks and broadband internet that provide computers and mobile devices with the precise time.
However, in recent years, scientists have developed optical atomic clocks, using a soft silvery-gold metal called caesium, that have far surpassed the accuracy of microwave clocks. Optical clocks are now the most precise clocks currently in existence.
"As clocks get more and more accurate, one thing that has been realised is that clocks change as you move in a gravitational field. If you move it up in the gravitational field, it moves a bit, so you could use it to map the world's gravitational field," Murray Barret, an associate professor with NUS' department of physics and a principal investigator with CQT explains.
It is currently very difficult to detect very small changes in gravitational fields with a high level of accuracy, but a better optical atomic clock could change that.
"We're exploring a completely different type of atom for clocks. We are using lutetium, a type of metal that is singly ionised. Single ions are easy to control. With ions, if we can go to many ions, we would have the ability to control the environment.
"This particular atom will have the right properties that lets us go to many ions, meaning it would have an impact on what technological devices you could make, for example chip-scale devices."
Quantum thermodynamics
Another important field of quantum technologies is quantum thermodynamics. Regular thermodynamics is the theory behind our understanding and design of thermal machines such as refrigerators and engines.
Engineers and scientists use statistical reasoning and some basic assumptions to predict how gases and fluids of different temperatures and pressures compress or expand, or how gases and fluids heat up or cool down when brought into contact with one another.
The concepts of thermodynamics work for large systems, but when systems are incredibly small, quantum effects also play a role, so key attributes like temperature, pressure and volume might not have the same meaning in small systems, like the nanoscale engines and devices being developed in laboratories around the world.
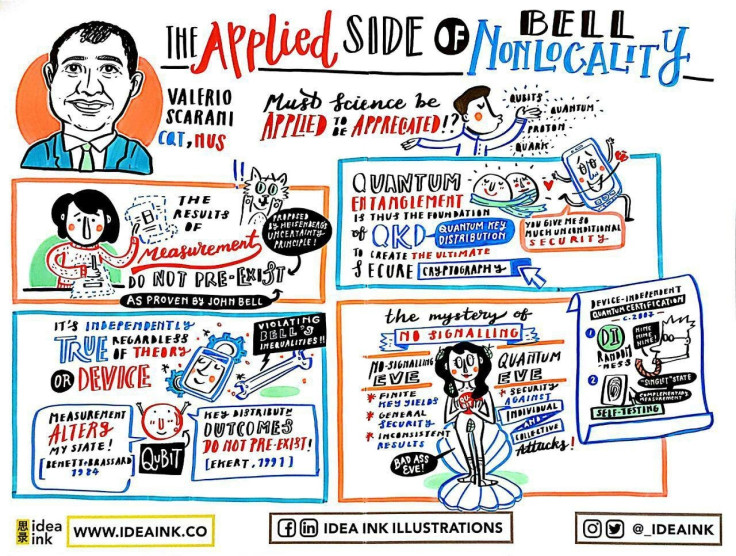
"We are reformulating and generalising the concepts of thermodynamics in the language of 'quantum information theory', a rapidly developing field that underlies our efforts to establish quantum computers, quantum communication, and quantum cryptography," explains Dr Stefan Nimmrichter, a research fellow at CQT.
"Here, the information content is treated as the main resource of a quantum system, which can be measured or lost to the environment. The laws of thermodynamics can be viewed as rules of book-keeping for that information content.
"Now that we start to understand the role of quantum effects in the thermodynamics of small systems, we want to know if we can use these quantum effects in systems to build a simple quantum engine or a fridge. It might be that it would behave better or more efficiently than a classical engine or fridge. If it's not possible, we need to know why."
Many of the nanoscale devices being developed in the world consist of single atoms interacting with a single quanta of light or mechanical vibrations in order to work.
But understanding quantum effects in thermodynamics is not just for building better systems – it can also help scientists to understand biological processes in nature better.
"Photosynthesis for instance, the way that plants collect energy from the sun, happens on the level of nano-sized molecular complexes – tiny machines that harvest energy quanta from sunlight and convert them into chemical energy and heat," says Nimmrichter.
© Copyright IBTimes 2025. All rights reserved.